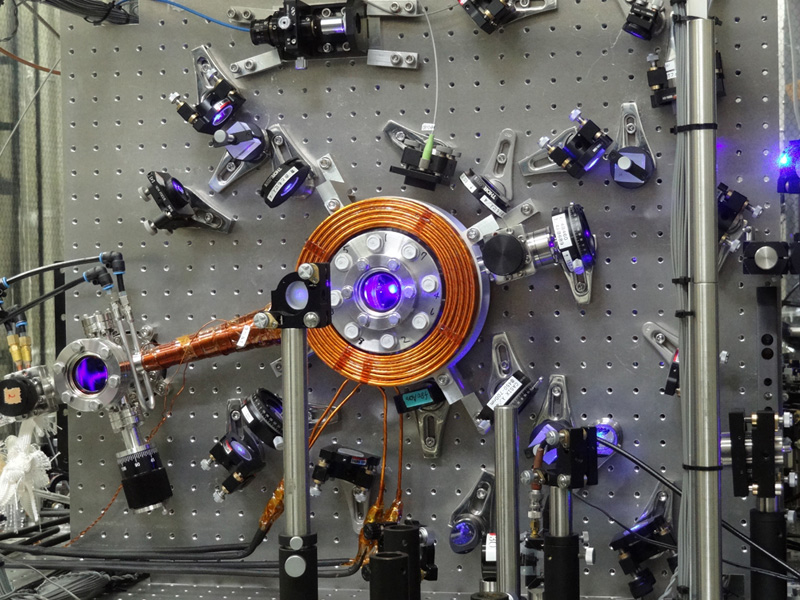
An optical lattice clock. A porthole with a diameter of 30 mm offers a glimpse into the ultra-high vacuum chamber, where approximately one million laser-cooled ultracold strontium atoms emit bluish-white fluorescence. Researchers trap these atoms in an optical lattice constructed by the Magic Wavelength, measure their resonant frequency, and use it as a clock pendulum. © Hidetoshi Katori
The first thing that entrance exam proctors do in the morning when they are summoned to duty is set their watches by the NTT (Nippon Telegraph and Telephone) time signal. If these are quartz watches with a monthly deviation of 30 seconds, they will remain accurate to within one second for the entrance exam on the next day. This performance is expressed with the equation 30s / 1 month = 30s / 2,592,000s (≒1/100,000). Such watches are referred to as 5-digit precision watches. The cesium atomic clock used for setting the NTT time signal has 15 digits of precision, and will remain accurate for 1015s (roughly 30 million years) before it loses even a second.
Clocks used to be tools for sharing time. The purpose of clock research is to find periodic phenomena as regular as possible and develop mechanisms to count them. The sundial may look quite primitive, but as a matter of fact, until the mid-twentieth century, time was defined astronomically based on the rotation of the Earth. The trouble was that tidal friction gradually slows down the Earth's rotation. Ironically, progress in astronomical observation revealed that the "astronomical second" was unfit to define time.
In 1955, the English physicist Louis Essen invented the cesium atomic clock. The idea was that if the second is defined using oscillations of atoms, the basic unit of matter, it would be possible to realize universal time. Just as ancient people believed that the motion of the Sun never changes, (many) physicists think that physical constants must be invariable quantities.
In 1967, the second was defined as the duration of 9,192,631,770 cycles of radiation corresponding to the transition between the two hyperfine states in the ground state of cesium atoms. A 10-digit significant figure sufficed in order to secure consistency with the "astronomical second" at the time. This "physical second" advanced with giant strides, and today is shared with 15 digits of precision. Car navigation systems, which determine a vehicle's location based on the observation of more than four GPS satellites by referencing their atomic clocks, are a modern version of the ancient celestial navigation methods. High-precision atomic clocks have already penetrated deep into our daily life.
The evolution of clocks is, in fact, the history of the speeding-up of the pendulum. The pendulum of Huygens, in which each swing takes one second, evolved into the quartz, which oscillates at a rate of 32,768 cycles per second. Next, scientists came up with the idea that if they could utilize the oscillations of light waves (several hundred trillion cycles per second), which are tens of thousands of times faster than the oscillations of the microwaves used in cesium atomic clocks, it would be possible to create atomic clocks of even greater precision. The invention of the laser, which is an oscillator at optical frequency, boosted development of an optical atomic clock.
In 1982, Hans G. Dehmelt proposed a single ion optical clock that measures the frequency of light absorbed by an ion captured in a Paul trap (quadrupole ion trap), and pointed out the possibility for development of an atomic clock with 18-digit precision. Since then, this method became the standard practice in the development of optical atomic clocks. In 2010, David J. Wineland, who back in the 1980s began research together with Dehmelt, finally enhanced the precision of the single ion optical clock to 17 digits. Wineland was awarded the 2012 Nobel Prize for Physics, thus becoming one of the 20 scientists together with Dehmelt and Wolfgang Paul who have received the Nobel Prize for research that contributes to the improvement of the performance of atomic clocks.
Strange as it may sound, it takes time to determine the accurate time. This is because of the quantum noise that appears when measuring the atomic states after exciting the clock pendulum. In single ion optical clocks, multiple averages are taken in order to reduce the quantum noise. As a result, it is necessary to repeat one million measurements over a period of approximately 10 days in order to read out an atomic clock with 18-digit precision. Conversely, fluctuations in the passage of time during the averaging, for example due to variations in gravity, will remain unnoticed. There are time domains ahead of us that we are still unable to access.
If we could find a way to measure one million atoms simultaneously, it would be possible to achieve 18-digit precision with a single one-second measurement. This is the vision that inspired the invention of the optical lattice clock. If we could confine atoms in a container that is invisible to atoms, atoms would absorb light at their resonant frequency even in this container. We found such container can be made by a laser tuned to a specific wavelength, which we named the Magic Wavelength. In the optical lattice clock, an egg-carton-shaped container for atoms is created using a standing wave of lasers tuned to the Magic Wavelength, enabling simultaneous measurement of one million atoms or more.
We conducted our first experiment in 2003, and since then optical lattice clock research has spread rapidly, due to the novelty and originality of this approach among other factors. In 2006, it was adopted as one of the "secondary representations of the second," which is a list of promising candidates for future redefinition of the second. In 2011, the International Committee for Weights and Measures recommended the transition frequency of optical lattice clocks that use strontium atoms to be 429,228,004,229,873.4Hz, whose uncertainty is restricted solely by the 15-digit realization precision of the SI second (one second in the International System of Units). This situation is similar to the circumstances in 1967 when Cs atomic clocks monitored the uncertainty of the "astronomical second." Today, the optical lattice clock has become a "super clock" that monitors uncertainties of the cesium atomic clocks. More than 20 research groups around the world are advancing development of optical lattice clocks targeting future redefinition of the second. In the near future, with the next changing of the guard, we will be able to share time to 18-digit precision.
The ability to read out 18-digit-precision time in real time will reveal relativistic space-time curved by the Earth's gravitational field. Such space-time curvature will be observable on the scale of our daily lives. Clocks, once perceived as a tool to share time with others, will assume a new role that is radically different from their conventional ones. By positioning an optical lattice clock even just 1 cm closer to the ground, we will be able to observe the slowing down of the passage of time due to the stronger gravity. Thus, clocks will transform into probes for exploring space-time distorted by gravity. A world view reminiscent of the "melting clocks" in the famous Salvador Dali's painting, The Persistence of Memory, inspired by Einstein's theory of relativity, will become a reality.
Do atomic clocks tick at a constant rate independent of the atomic elements used for the clocks? Yes, unless the physical constants undergo temporal and spatial changes. The new clocks will transcend their conventional function as a tool to share and confirm time, and will take on a new mission that defies the common sense of physics.
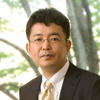