AI-assisted galactic observation unveils secrets of early universe
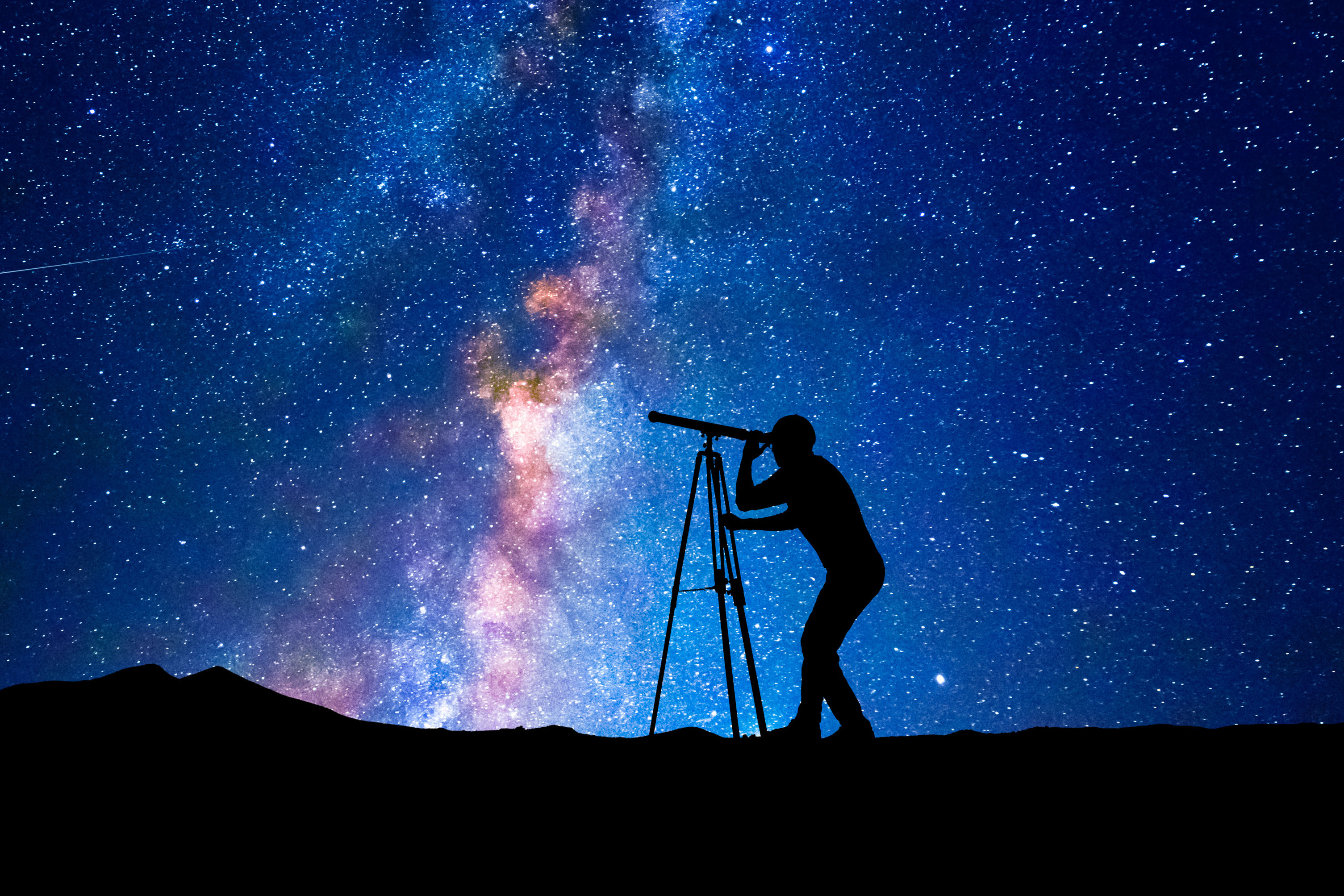
(Updated to the latest version on January 10, 2025 5:30PM)
Many scientists have found applications for the artificial intelligence approach known as deep learning, and astronomers are no exception. Kana Moriwaki, who is an assistant professor in the Research Center for the Early Universe, is one such researcher. Winner of the third Marie Skłodowska Curie Award Grand Prize, recognizing the achievements of early-career female researchers, Moriwaki explains what we can learn from observing galaxies.
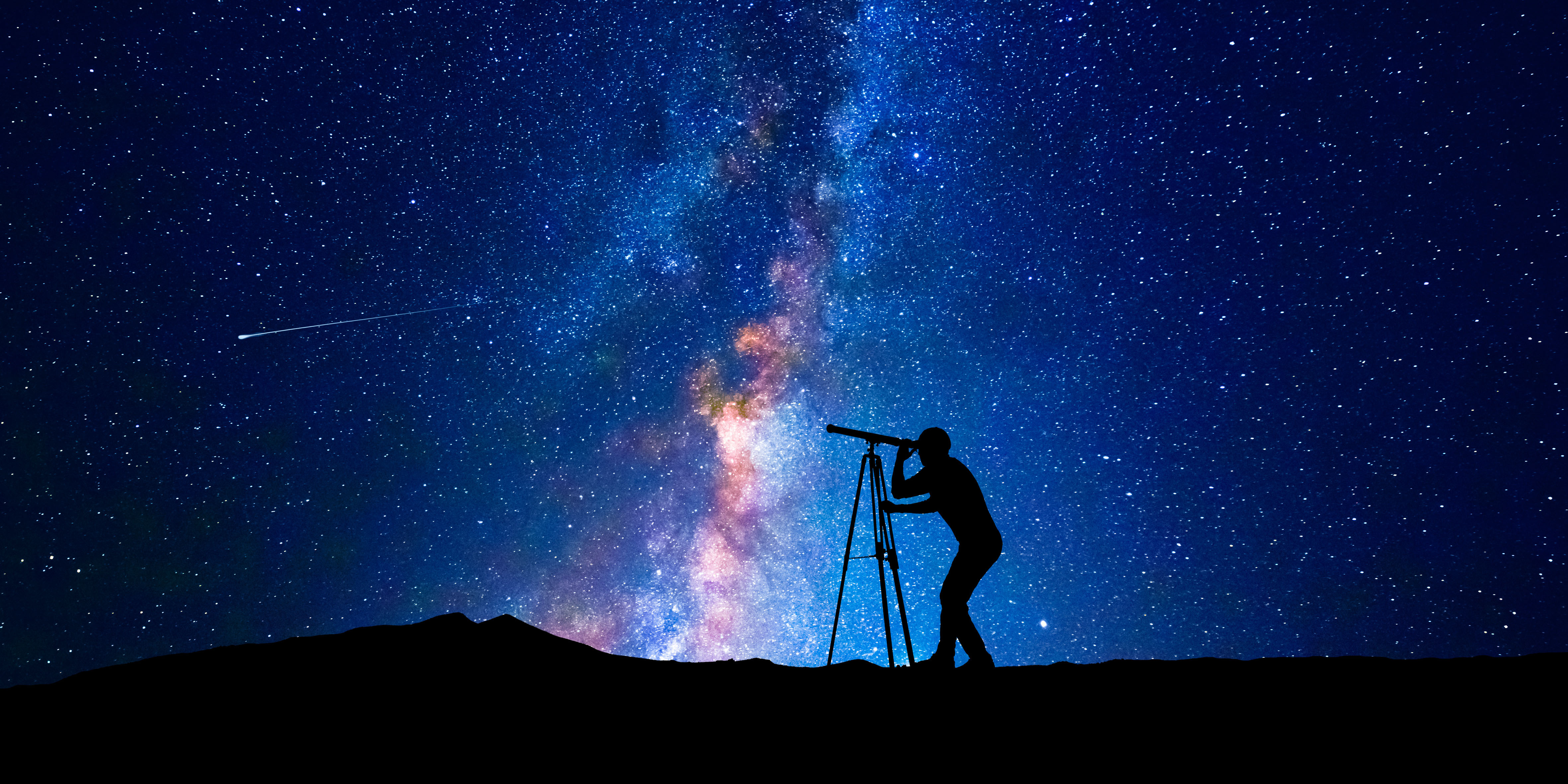
Looking into the past through faraway galaxies
── How much do we know about the evolution of the universe?
We know that the planet we live on, Earth, is located within the solar system and that our solar system is part of the Milky Way galaxy. We also know that there are many galaxies other than our own and the universe encompasses it all. By integrating various theories and the observational data that have been collected, we can form a rough idea of how the universe evolved.
Scientists say the universe was created around 13.8 billion years ago through a rapid expansion called inflation, followed by the Big Bang, the hot, dense rapidly expanding initial phase of the universe. After that, hydrogen gas and a mysterious substance called dark matter spread thinly throughout the universe, and space was devoid of celestial objects during this period. Then, at some point, stars were born, which then grouped together and formed galaxies, but the exact timing of these events is still unclear. For that reason, researchers have been trying to trace the history of the universe by observing more distant galaxies. Because it takes a long time for light from far-off galaxies to reach Earth, we cannot see what is happening there at this very moment, but we can instead see what they were like in the distant past. This is what allows us to learn about the history of the universe.
── How many galaxies are there in the universe?
While we do not know the physical size of the universe itself, we have been able to determine the limit of the observable universe based on the cosmic microwaves emitted after the Big Bang. That is to say, we know the farthest distance, or in other words how far back in the past, we can see. Within this range, even if we limit and include just the galaxies with a certain level of brightness that we can observe with current technology, there are still hundreds of thousands to a few million such galaxies. If we include fainter galaxies, you could say the number is nearly infinite.
The Atacama Large Millimeter/submillimeter Array (ALMA) radio telescope in Chile, which was constructed through an international partnership including Japan, contributed to the detection of the most distant observable galaxy to date, allowing us to see further into the past than previously possible. Radio telescope technology such as that used by ALMA enables us to see galaxies from approximately 13 billion years ago, only several hundred million years after the birth of the universe. More recently, the James Webb Space Telescope launched in 2021 by NASA, the U.S. space agency, discovered an even farther galaxy that dates from a mere 300 million years after the birth of the universe.
While ALMA and the Webb space telescope are used to discover and observe individual, far-off galaxies, I utilize next-generation telescope technology in my research to observe tens of millions of galaxies, forming a cluster, all at once.
Data science applications
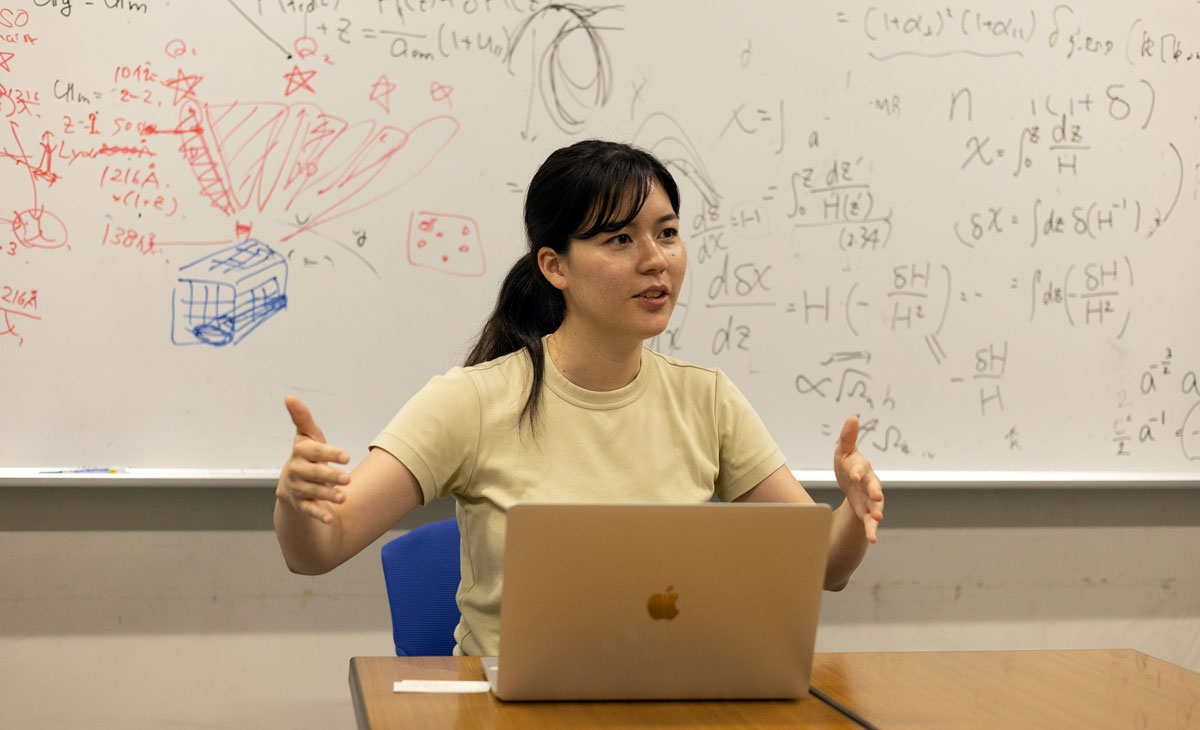
── What projects are you working on now?
I would like to understand when the universe began and when and how galaxies formed as a way of answering the fundamental question of why we exist in the here and now. We already have a lot of information about galaxies that formed about 1 billion years ago, but we are still trying to work out how galaxies that are several billion years old, or even older, were distributed throughout the universe. The more distant the object, the more difficult it is to observe; so in order to obtain clues, we first need to consider what kind of observation methods are appropriate, and then use theoretical simulations to infer how the presence of galaxies would impact the evolution of the universe as a whole. Ultimately, the goal is to understand the distribution of the galactic population, or in other words, map where each of the tens of millions of galaxies is located in the universe.
I expect to obtain observational data for my research from the SPHEREx near-infrared space observatory, which NASA plans to launch in 2025. I am also working with collaborators in Japan on a project to build photosensors capable of detecting millimeter-wavelength light. This is a joint effort in which our team makes theoretical predictions and proposes analysis methods, while space observation specialists build and operate the sensors. As it is difficult to manually analyze observational data of this volume, we are seeking to incorporate data science methods that will allow us to extract the necessary data to infer the large-scale structure of galaxies at certain cosmological eras.
── What exactly is involved in that process?
We are using an artificial intelligence approach known as deep learning, in order to extract what we need from the captured two-dimensional and three-dimensional image data. This is because when the data is initially captured, it contains a lot of unnecessary information, known as “noise.” This led us to create a deep learning model that automatically removes that noise by drawing on deep learning models that are used for image editing. There are image processing techniques, developed through research in the information science field, that can, for example, separate the indoor elements of a photograph taken through a window from inside a room, and extract just the outdoor scene beyond the window. The application of this technology to the field of astronomy has made it possible to remove various types of noise, such as atmospheric fluctuations and images of our neighboring galaxies, that turn up when observing far-off galaxies from Earth. When scanning for light at specific wavelengths, known as emission lines, other wavelengths of light emitted by galaxies also become noise, so it is necessary to remove those as well.
In addition to radio waves, in astronomy we also make observations using various other means, including visible light, which we can see with the naked eye, and infrared and ultraviolet light, which are not visible to humans. When we focus on a galaxy’s particular emission line, the wavelength of that line correlates to the distance from Earth to that galaxy, or in other words, it corresponds to the era at which the light was first emitted from that galaxy. In addition to the distribution of light on the celestial sphere (an imaginary sphere used to map objects in deep space), I also investigate emission line wavelengths to determine the galaxies’ distribution in three-dimensional space. I anticipate realizing this goal within a few years with the help of SPHEREx, which I mentioned earlier.
The parameters that define our existence
── What can we expect to learn as observation technologies improve?
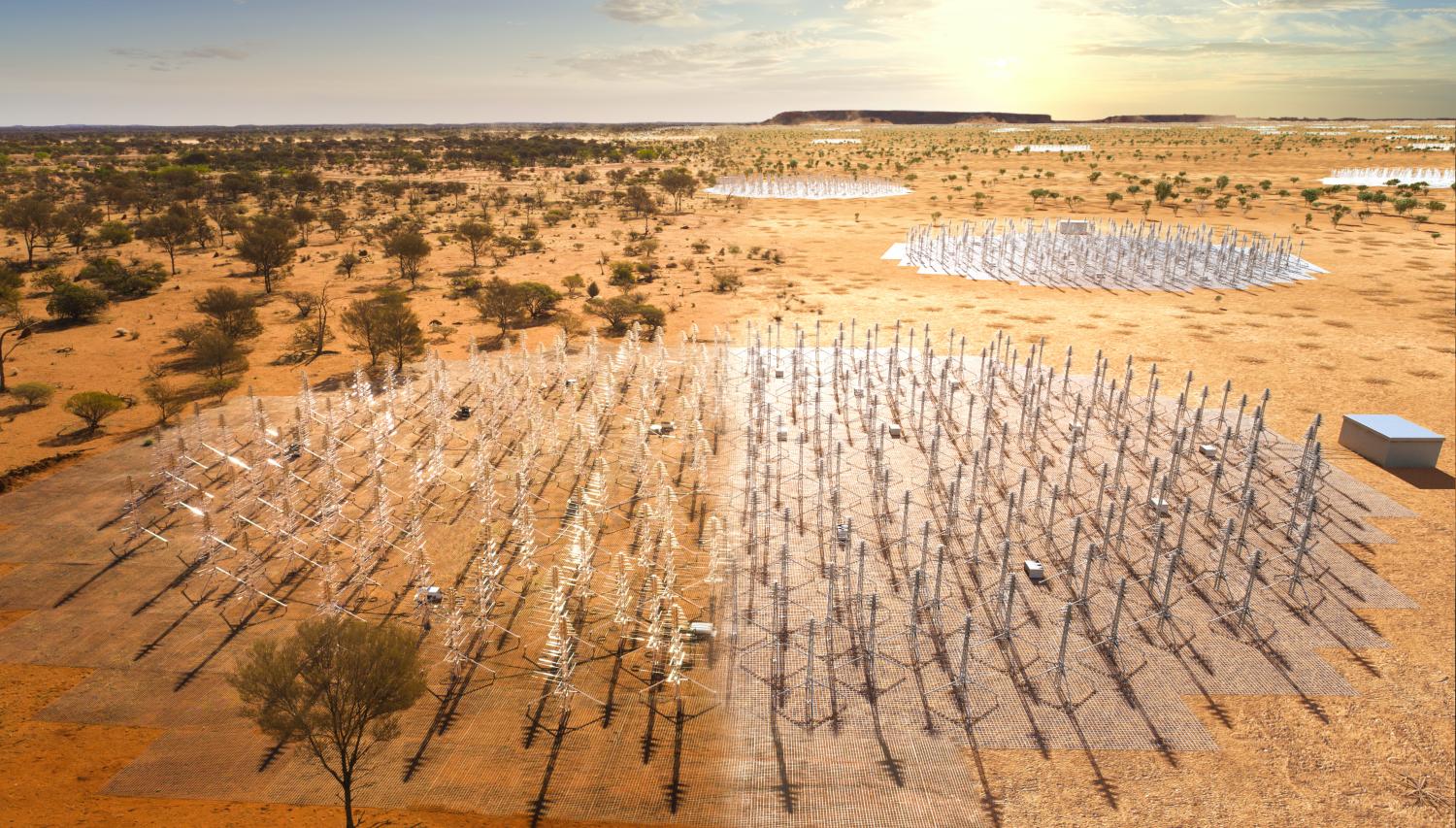
Space telescopes like Webb have advanced research on the evolution of the universe. However, although the Webb telescope can detect and observe individual galaxies, it cannot provide a bird’s-eye view of how galaxies are scattered over a wide area. This is where SPHEREx and the submillimeter-band sensors we are developing — which we plan to attach to the Atacama Submillimeter Telescope Experiment in Chile — will come into play.
In the future, the technologies that allow for the observation of space without atmospheric or radio wave interference, such as the lunar observatory envisioned by JAXA (Japan Aerospace Exploration Agency), are expected to improve. There is also a method for ground-based observation with radio waves that involves arranging many antennas on a large swath of land. The Murchison Radio-astronomy Observatory in Western Australia plans to begin operating one such project in 2028, in which antennas are densely packed in an area the size of a square measuring 1 kilometer across. Deserts, like where this observatory is located, are ideal for radio wave observations because these remote areas are devoid of interference by the artificial radio waves generated by humans, which have become part and parcel of our daily lives.
Such advances in various observational technologies may provide clues not only about the nature of the current universe, but also of the primordial universe. At the beginning of the universe during the Big Bang, protons and electrons moved about in an ionized plasma state (a phase of matter distinct from solids, liquids and gases). The particles then entered a neutral, nonionized state. However, current research suggests that after some time, the protons and electrons in the gas spread throughout the universe actually reionized.
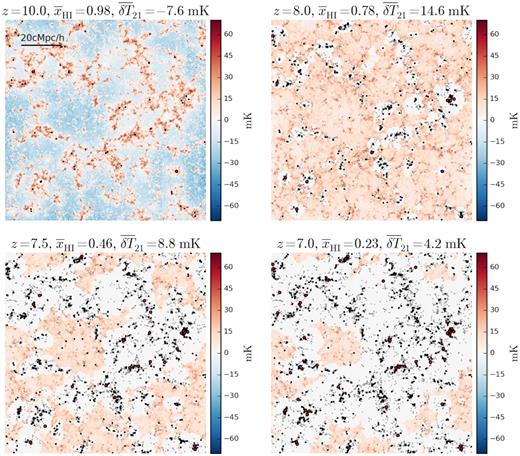
Source: Moriwaki, Kana, Naoki Yoshida, Marius B Eide, & Benedetta Ciardi. (2019). “Cross-correlation between the 21-cm signal and [O iii] emitters during early cosmic reionization.” Monthly Notices of the Royal Astronomical Society, vol. 489 (2), pp. 2471–2477. DOI: https://doi.org/10.1093/mnras/stz2308
Elucidating when this epoch of reionization occurred is critical for understanding the early universe, and examining far-off neutral hydrogen gas and oxygen ions may provide some clues. Neutral hydrogen gas, which is spread thinly throughout the universe, is dark and difficult to see, but it glows and is seen as a more intense line in a spectrum at the radio wavelength of 21 centimeters. However, analyzing this faint light requires that we combine it with observations of emission lines in other wavelengths, such as that of oxygen ions. Although ultraviolet light has received much of the attention so far, our group was the first to demonstrate that the observational data of visible light and far-infrared light emitted from oxygen ions are also useful for a better understanding of cosmic reionization more broadly. Going forward, my hope is that radio wave observations will yield more information on how galaxies heat and ionize neighboring gases.
── What is something you were able to understand differently thanks to research on the universe?
One major thing is the fact that the mystery of why the universe came into existence is directly connected to the question of why we exist in the here and now. Our Earth, with an environment that supports human life, only exists, sustained by the Milky Way and stars like our sun. If the history of ionized gas expansion throughout space had been different, the galaxy in which we live may have looked completely different today. In cosmology, the numerical values that describe the nature of the universe, such as the percentage of dark energy, are called cosmological parameters. If these parameters had been different, galaxies may not have formed, and we might not even exist today. When you think about it that way, the study of astronomy can raise some very philosophical questions.
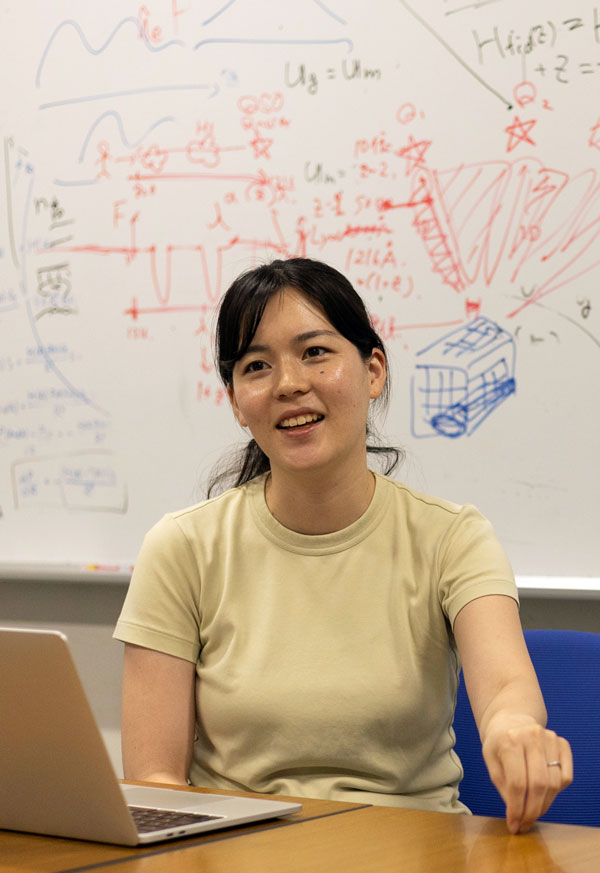
Kana Moriwaki
Assistant Professor, Research Center for the Early Universe
Ph.D. from the Department of Physics in the Graduate School of Science at the University of Tokyo. Conducts research on galaxy formation and evolution, cosmic reionization and cosmology using cosmological simulations and machine learning. Assumed current position in 2022. Awarded the third Marie Skłodowska Curie Award Grand Prize, co-organized by Japan Science and Technology Agency and the Embassy of the Republic of Poland in Tokyo, in 2024. Author of Large-Scale Structure of the Universe – Cosmological Simulations and Machine Learning (Springer Singapore, 2022), and co-author of numerous journal articles, including Moriwaki, Kana, et al. “Deep-learning Reconstruction of Three-dimensional Galaxy Distributions with Intensity Mapping Observations” (The Astrophysical Journal Letters) and “Cross-correlation between the 21-cm signal and [O iii] emitters during early cosmic reionization” (Monthly Notices of the Royal Astronomical Society), among others.
Interview date: July 11, 2024
Interview: Yuki Terada, Hannah Dahlberg-Dodd