Why do recordings of one’s own voice sound so strange?
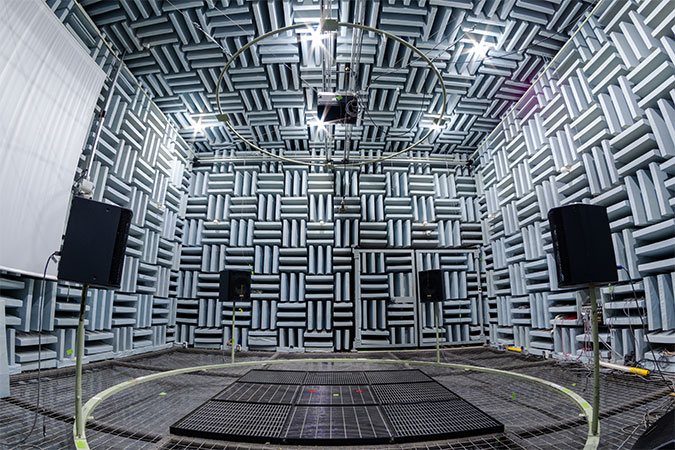
An introduction to academia starting with “Why?”
After making a list of questions which would pique anyone’s interest, we asked faculty members on campus who might be knowledgeable in these areas to answer them from the perspective of their respective expertise. Let’s take a look into the world of research through questions that you feel you know something about, but cannot answer definitively when actually asked.
Q11. Why do recordings of one’s own voice sound so strange?
Many people are bewildered when they hear their own voice in recorded form. Why does it sound so different from our own perception of our voice?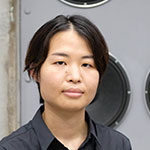
Research Associate, Institute of Industrial Science
Architectural Environmental Engineering |
The difference between “air-transmitted sound” and “bone-transmitted sound”
Our ears receive sound via two different routes of transmission: vibrations in the air reaching the eardrum (air-transmitted sound) and vibrations in our bones (bone-transmitted sound). Usually you hear your own voice through a mixture of sounds received through both these routes. When you hear a recording of your voice it sounds different, because it comes only in the form of air-transmitted sound. This air-transmitted version of your voice is the same as what other people hear when you speak.
All the external sounds that we hear, whether it be other people’s voices, music or noise, reach us in the form of vibrations transmitted through the air. These vibrations enter our ear through what’s known as the ear canal, and cause the eardrum at the end of the canal to vibrate. These vibrations travel through three bones known as the auditory ossicles, which lie even deeper in our ear, and enter the cochlea, an organ shaped like a snail’s shell. The cochlea converts these vibrations into electrical impulses, which are then transmitted to the auditory nerve. This is how air-transmitted sound works. For bone-transmitted sound, the vibrations travel through the temporal bone (one of the bones in our skull) directly to the cochlea. You can still hear your own voice even when you cover your ears. That is because you’re hearing it mainly as bone-transmitted sound.
Human ears are less sensitive to low frequency sounds
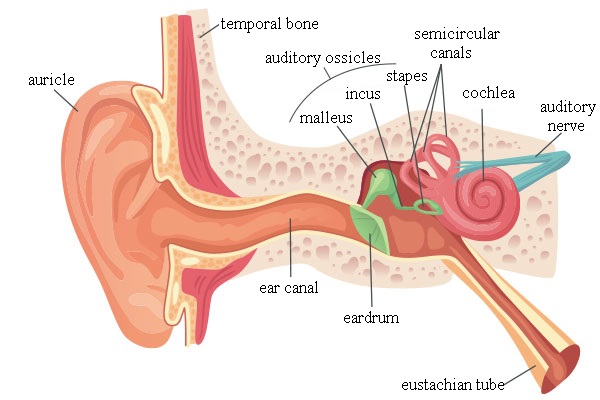
My research on sound focuses on acoustics in architectural environmental engineering. The word “environment” might bring to mind planetary-scale environmental issues such as global warming, but my research deals with the acoustic environment, which is on a much more familiar scale: a radius of around five meters up to a maximum of 100 meters. Our living environment is full of sounds from household appliances, air-conditioning and many other sources. I’ve been tackling questions related to noise pollution arising from this environment, such as what kinds of sounds are generated and why, and how well humans can tolerate them.
Humans are said to be capable of detecting sounds in the frequency range from 20 to 20,000 hertz (Hz). This unit of frequency represents how many times the air vibrates each second. The fewer Hz, the lower pitched the sound is; the more, the higher. For example, the hum of a refrigerator is a low frequency sound at around 50 Hz, and cooling fans in computers sometimes make strong, high-pitched whirring sounds that can reach frequencies of over 1,000 Hz. Such sounds can be unpleasant even if they are not loud. I conduct experiments that evaluate sounds in terms of how unpleasant they are, ascertaining differences in the psychological degree of discomfort felt by listeners based on detailed analysis of physical characteristics of each sound such as frequency and intensity.
Among all these sounds that we live with, I’m especially interested in low frequency sounds. One of the characteristics of human ears is their insensitivity to low frequency sounds. When calculating noise indicators, which are widely used in guidelines such as the environmental quality standards for noise issued by Japan’s Ministry of the Environment, the energy of low frequency sound is often accounted for with a low weighting due to the sensitivity of the human ear. This is not a problem in ordinary noise situations, but low frequency sounds that are especially noticeable can affect the overall sound quality and be a source of discomfort. This is a problem I have been seeking to address.
For example, external air-conditioning units sometimes produce sounds in a low frequency range of around 50 to 100 Hz. When they run all night they can interrupt your sleep and disturb your neighbors. One important step in tackling this noise pollution problem is to measure quantitatively what frequency and strength of sound causes people discomfort. I believe that if we can ascertain what levels can be tolerated, we’ll be able to find ways to deal with these sounds, such as proposing target values for limiting noise pollution at the product development stage.
Creating a comfortable acoustic environment
The ultimate aim of my research on noise pollution is to eliminate unpleasant sounds and create a more comfortable acoustic environment. These days it’s possible to reduce the unpleasant sounds that reach your own ears by using noise-cancelling earphones, but from the viewpoint of architectural acoustics, we want to make more spaces comfortable without the need to use such devices. One example is railway stations in Japan. Railway stations have many noise sources like the clickety-clack of trains and crowds of people. In addition to these noise sources, numerous announcements are broadcast, such as safety messages on escalators and train arrival and departure announcements. Such noisy environments, in turn, force people to talk in louder voices, creating an extremely noisy assortment of sounds. Announcements are essential for information transmission and the safety of users, but I believe we can create a quieter environment through noise reduction strategies such as modifying the approach to announcements and selecting appropriate building materials.
Sounds are invisible in architectural drawings, and therefore tend to receive low priority. But I want people to feel how much more pleasant spaces can be when they are a little quieter, and I want to communicate the importance of acoustics to those who design and make decisions about how spaces are built. Acoustic environments can be altered by changing the shapes and materials of our buildings. There are also real-life considerations such as cost, but I believe it’s important to give more thought to acoustical environments.
We still know very little about how humans perceive sound holistically, and what factors affect our judgment of the quality of an acoustic environment. For example, what frequency characteristics does a sound have, and how does it change over time? Or, why can sounds with identical physical properties have a different impression on you depending on whether they’re made by a friend or by somebody you don’t know? There are many different routes by which we evaluate our acoustic environment depending on the circumstances, and I hope to be able to shed light on at least one or two of these routes.
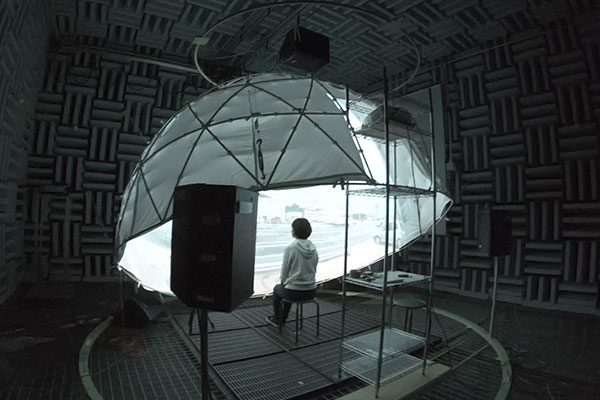
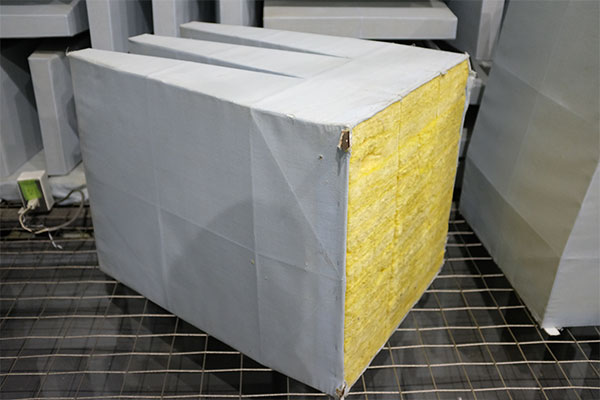
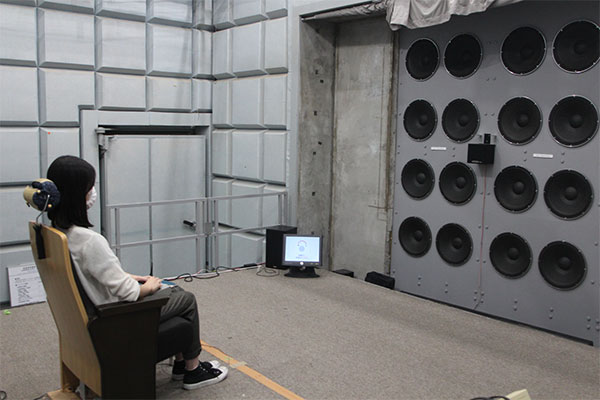
* This article was originally printed in Tansei 45 (Japanese language only). All information in this article is as of September 2022.